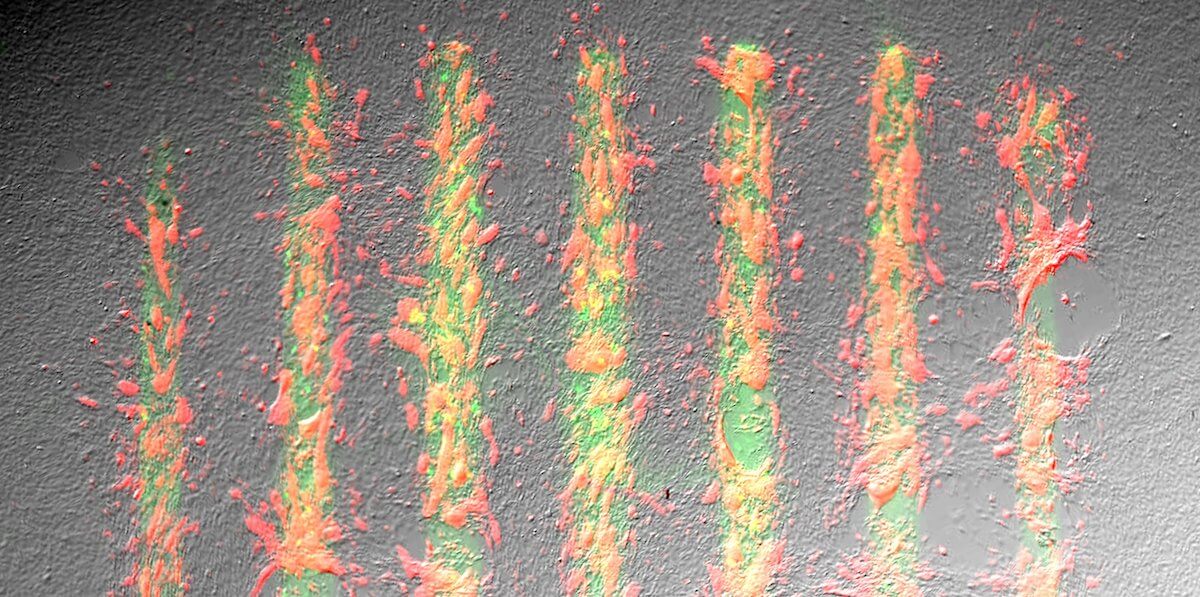
It may sound like something from science fiction. To grow and modify muscle tissue in the lab using technology that shapes the muscle cells, blood vessels and nerves into whatever pattern you need. A new major project supported by a Chan Zuckerberg Initiative (CZI) grant will help bring this technology to fruition, offering revolutionary potential for treating neuromuscular disorders and other diseases.
The research team is led by Megan McCain, an associate professor of biomedical engineering at the USC Viterbi School of Engineering, and Leonardo Morsut, assistant professor of stem cell biology and regenerative medicine at the Keck School of Medicine of USC.
The work is part of a collaborative $4 million project with the California Institute of Technology and the University of California, Los Angeles, that will collectively develop and apply new technologies to explore how cells interact. The project is funded through CZI’s Exploratory Cell Networks grants to support early-stage technology development driven by collaborations across regional labs.
McCain said that the biomedical community has spent decades creating a range of methods to convert stem cells into different classes of cells, from muscle or nerve cells to immune or blood vessel cells.
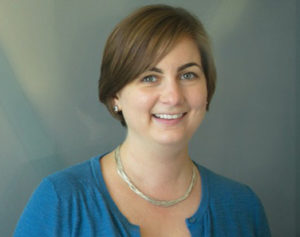
“But it’s something that is always done in isolation,” said McCain, the Chonette Early Career Chair in the Alfred E. Mann Department of Biomedical Engineering. “You make all your muscle cells in one dish, you make all your neurons in another dish and then you mix them together to make a tissue. But that’s not how things happen in the embryo, where all these cells are developing at the same time, right next to each other.”
This process of creating different cell types in separate laboratory environments also has disadvantages, McCain said, because it doesn’t allow users to control where and how the cells will grow. The research team aims to mimic natural development more closely, which could create more mature tissues that enable researchers to control the structure and shape at a cellular level.
“There are few technologies that exist today to make precise tissues at the level of a single cell,” McCain said. “We’re trying to engineer tissues in a very precise way by dictating the identity and pattern of individual cells.”
Training cells to grow how and where you want them
McCain’s Laboratory for Living Systems Engineering has been working closely with Morsut, a synthetic biology expert, who also holds a courtesy appointment in the Mann Department of Biomedical Engineering.
Morsut and his team treat cells like computers, reprogramming them with synthetic receptors.
“We insert a new set of instructions in the cell’s operation program (the genome),” Morsut said. “One set reads as: ‘When you touch a green fluorescent protein, start the program to become a muscle cell.’”
Meanwhile, McCain’s lab creates materials and scaffolds that work like customized building blocks to control tissue patterning. In this project, for example, they pattern scaffolds with proteins, such as green fluorescent protein, to activate the synthetic parts of the Morsut lab’s cells – notably, the receptors on the outside of the cells.
“And all this is modular,” Morsut said, “so we can swap the program with one that says: ‘When you see a red fluorescent protein, start the program for endothelial (blood vessel) cells.’ So now, if we have all these new instructions in one cell, and we pattern their growth environment with carefully placed signals (green or red fluorescent proteins), then we can control the architecture of the different cell types.”
The scaffolding that McCain and her colleagues are building will also allow researchers to control the three-dimensional growth of the tissue precisely.
The project will also involve a series of sub-collaborations. Eun Ji Chung, USC Viterbi associate professor of biomedical engineering and Dr. Karl Jacob Jr. and Karl Jacob III Early-Career Chair, will work with the duo to develop muscle-tendon-bone tissue. Peter Yingxiao Wang, the Dwight C. and Hildagarde E. Baum Chair in Biomedical Engineering, will also work with the team to control cell identity in tissues by shining different wavelengths of light on them. The team will also collaborate with Matt Thomson, a Caltech assistant professor of computational biology, and Tom Rando, UCLA professor of neurology, molecular, cell and developmental biology.
A toolbox to enable future breakthroughs
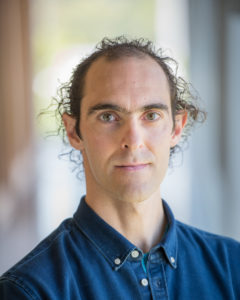
The result of the project will be a modular, biomedical toolbox that researchers and clinicians can harness to create tissues that could be game changers for regenerative medicine, drug discovery, neuromuscular disorders, and more.
“In theory, there could be endless possibilities for regenerative medicine, such as replacing not only muscle but also heart, kidney, liver, etc., as we get sick or old,” McCain said.
Another critical application is organ-on-chip systems, a specialization for McCain’s lab, in which tissues are grown on microfluidic chips to mimic human physiology. Organ-on-chip systems allow researchers to model diseases and measure human responses to drugs and therapies. One aim of the project will be modeling the neuromuscular junction — the crucial intersection between our motor neurons and muscle fibers. Improving our understanding of this connection will enable better treatments of neuromuscular diseases and disorders such as ALS and muscular dystrophy
“We also want to build tissues using cells collected directly from patients. Based on their genetic background, or if they have a certain genetic disease, we could make personalized neuromuscular tissues and screen drugs that may be more effective for those patients,” McCain said.
The project also aims to boost our general understanding of human tissue development. The precision engineering of tissues can give researchers a clearer insight into how cells communicate with each other during early development and at the embryonic stage.
“Controlling how to grow organs in the lab would be transformative for two goals: disease modeling in vitro and down the line transplantation of replacement organs,” Morsut said. “This will require two main advancements: new technologies to control cell behaviors in space and time and understanding the biology enough to be able to control it.”
McCain said the CZI investment is exciting as it promotes cross-institution collaboration and takes a risk with new technology that could potentially enable a wide range of applications and discoveries.
“CZI is interested in funding new technologies that are interdisciplinary and have potential for broad impact, which is a great fit for our project. We are excited and grateful for their investment in our collaborative project,” McCain said.